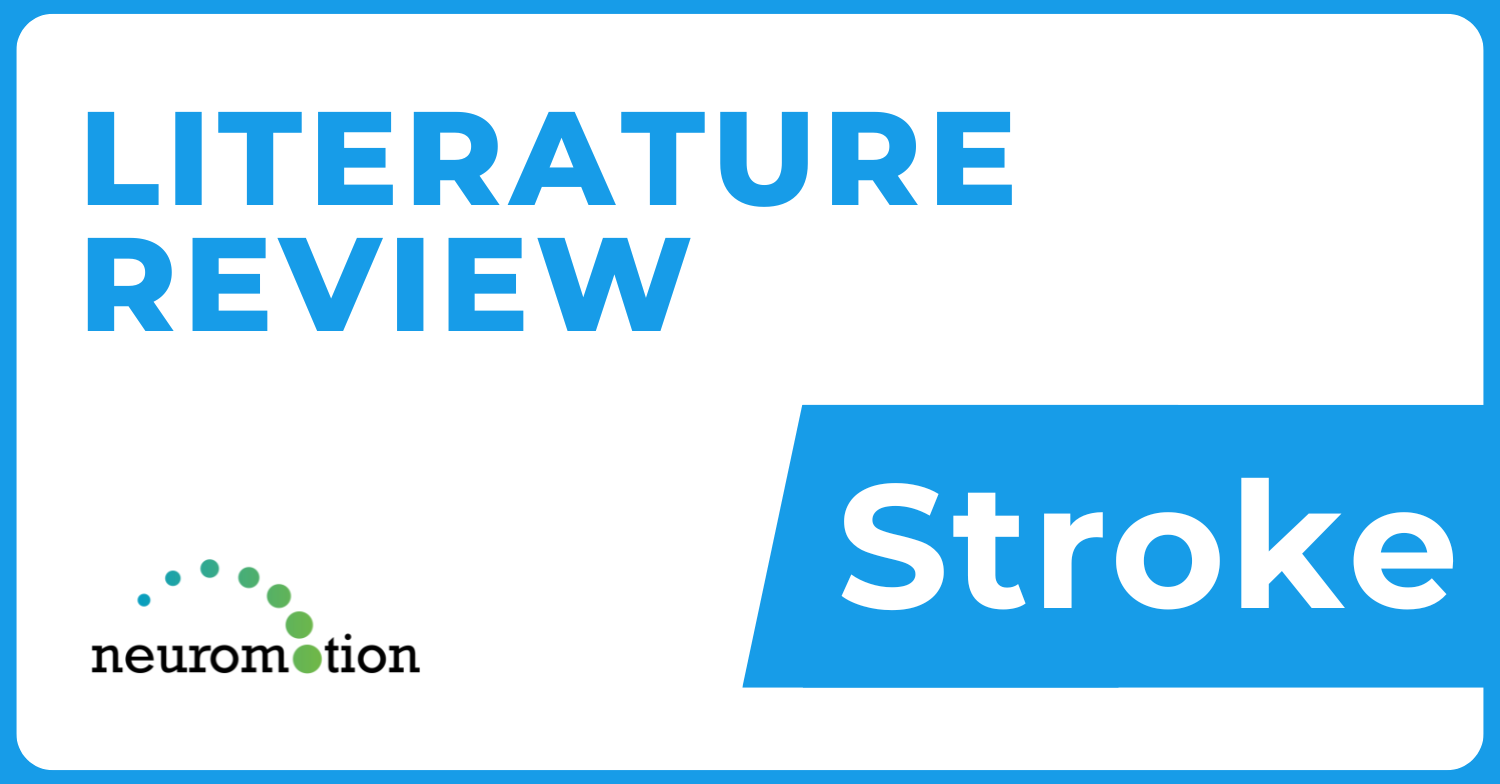
Background of Stroke
Stroke is seen to affect almost 80 million people worldwide. Stroke often leads to motor deficits, which are often presented in 80% of acute cases and 40% of chronic cases (Cabral, et al., 2022, p. 74). After stroke, there is a possibility for spontaneous recovery of function, but research shows this to only occur less than 15% of the time. The products of stroke, such as motor deficits, are strongly linked to mechanisms of neuroplasticity (Cabral, et al., 2022, p. 73). By doing rehabilitative therapies, these mechanisms of neuroplasticity can be used to recover functionality (Cauraugh & Summers, 2005, p. 309).
Risk Factors for Stroke (Murphy & Werring, 2020, pp. 652-653)
- Age – the risk doubles for each decade after the age of 55.
- Sex – premenopausal women have a higher incidence rate than men of the same age, due to the risks of pregnancy and oral contraceptives. At older ages, however, the risks for men are higher than that in women of the same age.
- Genetics – some genetic disorders are associated with an increased risk for stroke, e.g. Fabry’s disease, homocystinuria, sickle cell disease, and connective tissue disorders. Furthermore, the MEGASTROKE Consortium identified 22 novel loci in the human genome that are associated to stroke mechanisms.
- Hypertension (high blood pressure)
- Diabetes mellitus – doubles the risk of stroke
- Cardiac factors – e.g. atrial fibrillation
- Smoking - doubles the risk of stroke
- Substance and alcohol abuse
- Hyperlipidaemia (high levels of cholesterols and tiglycerides)
- Obesity
- Sedentary lifestyle
- Inflammation
- Infections – e.g. covid-19
Pathophysiology
EEG and functional-MRI studies have shown that stroke induces a change in the brain’s ability to process activity for movement, possibly as a compensatory strategy. However, this reorganization of brain activity is linked to the decreased motor function observed after stroke (Murphy & Werring, 2020, p. 9).
When making a prognosis for overall recovery after a stroke, the rate of recovery plays a great role. Research shows in the case of upper extremities, for example that voluntary movement should be observed in the upper extremities after 15 days post-stroke and measurable grip should be observed at 1-month post-stroke for a good prognosis of functional recovery in the arm. In the case of the lower extremities, hip movement at 1-week post-stroke is associated with a good prognosis for future ambulation (Good, et al., 2011, p. 545). When it comes to aphasia (loss of language) and dysarthria (disorder of speech), intense speech and language therapy in encouraged and is associated with good recovery within 3-6 months (Good, et al., 2011, p. 546)
Other Effects of Stroke
- Dysphagia (partial loss of language) – affects 42%-80% of stroke survivors (Good, et al., 2011, p. 558)
- Urinary incontinence (loss of bladder control) – affects 38%-60% of stroke survivors during early recovery (Good, et al., 2011, p. 560)
- Shoulder pain – affects 80% of stroke survivors (Good, et al., 2011, p. 560)
- Complex regional pain syndrome – affects 25% of stroke survivors, and presents as pain and oedema in the hand and shoulder. This can be treated with corticosteroids and anti-inflammatories (Good, et al., 2011, p. 560)
- Falls – 40% of stroke survivors experience falls in their course of rehabilitation
- Depression – affects up to 80% of stroke survivors, which affects their recovery (Good, et al., 2011, p. 561)
- Post stroke cognitive impairments (PSCI) – affects up to 80% of stroke survivors (Mane, et al., 2020, p. 2).
New research on stroke pathophysiology:
In researching the pathophysiology of stroke, researchers hope to identify the mechanisms of stroke which could ultimately become treatment targets. Recent research has found that the pathophysiology of stroke is linked to energy metabolism and mitochondrial dynamics in the brain (Zhou, et al., 2021, p. 1). The mitochondria are not just responsible for generating energy, but also drive active and passive cell death and thus play an active role in cell death after stroke (Zhou, et al., 2021, p. 3). During a stroke the normal glucose and oxygen supply to areas in the brain is interrupted, which alters the dynamics of mitochondria (e.g. altering the rates of mitochondrial division and fusion) in nerve cells thus impacting neuronal survival (Zhou, et al., 2021, p. 5). The altered dynamics of mitochondria leads to calcium overload, which induces the death of nerve cells. Further, reactive oxygen species levels increase due to mitochondrial dysfunction which further induces oxidative stress and tissue damage in the brain (Zhou, et al., 2021, p. 6). With this knowledge, researchers identified that neuronal death during stroke could be treated by interrupting these tissue damage cycles and inhibit neurotoxicity through regulating mitochondrial dynamics (Zhou, et al., 2021, p. 7).
Pharmacotherapy
Stroke treatment is often time sensitive, as in the case of tissue plasminogen activator (t-PA) for thromboembolic strokes which has to be administered within the first 4.5 hours of a stroke (Mohamadpour, et al., 2019, p. 2). Further, not many pharmacotherapies exist for treating stroke, however researcher are actively searching for solutions through clinical trials, such as the following (Zhou, et al., 2021, p. 9):
- Hyperbaric oxygen treatment to reduce brain damage
- Propofol to reduce the death of nerve cells and to alleviate nerve cell damage
- Atractylenolide III and AG490 (an inhibitor of jak2) therapy to treat middle cerebral artery occlusions (stroke) has shown promising results in attenuating brain swelling and neurological deficits in mouse models.
- Melatonin therapy is seen to promote mitochondrial fusion, inhibiting nerve cell death, reducing infarct size, and reducing reperfusion stress after cerebral hypoxia (stroke) in mouse models.
- 4-chloro-N-(naphthalen-1-ylmethyl)-5-(3-(piperazin-1-yl) phenoxy) thiophene-2-sulfonamide (B355252) treatment is being studied as a possible treatment to reduce reactive oxygen species and to maintain mitochondrial dynamics, thus to inhibit and reduce nerve cell death.
- miR-7 mimic oligonucleotide injection to reduce brain injury through inhibiting mitochondrial fragmentation, oxidative stress and neuronal cell death after cerebral ischemia (stroke).
- Studies have also shown that Nitric oxide synthase (NOS3) inhibition protects the mitochondrial structure and movement, and prevents mitochondrial division, thus preventing neuronal death. Granulocyte-colony stimulating factor(G-CSF) was used in mouse models and maintained cellular homeostasis in the brain and acted as a neuroprotectant. Photobiomodulation therapy is a new therapy which reduces nerve cell death in neonatal hypoxic-ischemic encephalopathy (a form of stroke in infants). The transplantation of mitochondria is also seen to reduce brain damage and cell death in rat models.
Physical Therapies
Traditional rehabilitation after stroke was mostly focussed on practicing and improving the effected skills. New rehabilitation protocols focusing on neuroplasticity are seen to enhance recovery (Norman, et al., 2022, p. 1). Neuroplasticity is necessary for post brain injury recovery, and is thus utilized by physiotherapy strategies which combine variable environments, learning, memorization and physical activities (Dabrowski, et al., 2019, p. 5). The type of rehabilitative training is however very important as it can determine the degree of neural plasticity (Cauraugh & Summers, 2005, p. 309). New research aims to use this concept as part of neuroprotective and regenerative therapies (Dabrowski, et al., 2019, p. 2). Thus, therapeutic strategies based on the concept of neuroplasticity can encourage significant improvements in the performances affected by injury (Dabrowski, et al., 2019, p. 5).
Neuromotion specifically incorporates the following principles of neuroplasticity into their stroke rehabilitative programs to enhance recovery in stroke survivors:
- Specificity: Rehabilitation needs to be targeted to the specific areas of your brain. Our therapists design individualized treatment plans based on initial assessment findings.
- Salience: Rehabilitation should focus on the things important to you. Our treatment plans focus on functional therapy and improving activities of daily living and recreational activities important to you.
- Difficulty: Therapy must be at an appropriate level of challenge and frequency of practice for neuroplasticity to take place. Our clinicians are trained to provide this level of challenge while keeping you safe.
- Intensity: Our program starts with a high frequency of sessions per week to ensure we are achieving the intensity required.
- Repetition: Repetition of a learned skill or movement is required to make long term changes in the brain. Research has shown that up to 2000 repetitions for one joint is required for neuroplasticity to take place and a functional improvement to occur. In addition, it can take up to 60 days for neuroplasticity to begin, therefore rehabilitation requires a minimum of 8 weeks of commitment.
Some therapeutic protocols encouraged by research include the following:
- TNP (targeted neuroplasticity protocol) creates an interaction with the central nervous system which creates plasticity at applicable neural pathways, ultimately improving function (Norman, et al., 2022, p. 6). Ideally, TNP therapy can induce adaptive plasticity, which reorganizes the neural network and enhances function (Norman, et al., 2022, p. 11).
- Bilateral training is encouraged for rehabilitative training as it facilitates neuroplasticity by means of increasing the use of the remaining neural pathways in the affected area of the brain; and by increasing communication between the hemispheres of the brain (Cauraugh & Summers, 2005, p. 309). Activity-dependant and bilateral symmetrical activities are encouraged for stroke rehabilitative therapies as it encourages the activation of both hemispheres of the brain and thus promotes plasticity (Cauraugh & Summers, 2005, p. 312).
- VNS (vagus nerve stimulation) is a treatment induced by implanted contacts which stimulate the vagus nerve and encourages motor recovery. Recent research has shown that VNS could also be used for cognitive enhancement and sensory restoration. Furthermore, methods are being explored to make this treatment less invasive (Saway, et al., 2024, p. 2).
- DBS (deep-brain stimulation) is an invasive treatment where an electrode is placed in the targeted area of the brain and then stimulates the target. This treatment has also shown to alleviate post-stroke pain (Saway, et al., 2024, p. 3).
- BCIs (brain-computer interfaces) provide computer assisted restoration of motor, sensory, and/or cognitive functions in individuals with severe neurological impairment (Saway, et al., 2024, p. 4). These devices receive and decode activity from the motor region of the brain and consequently give sensory-motor feedback through moving the respective hemiparetic limb, or controls a prosthesis, or gives visual feedback (Mane, et al., 2020, p. 2). The use of this technology in combination with physical and occupational therapy has shown promising results in stroke treatment, and is currently being further developed to assist in speech restoration (Saway, et al., 2024, p. 4).
- Constraint-induced movement therapy is a forced-use intervention where the stroke survivor is forced to use their paretic limb in movement exercises. This method has been associated with consistent functional motor gains (Cauraugh & Summers, 2005, p. 311) and changes in functional-MRI results suggests that this type of therapy induces reorganization in the brain (Good, et al., 2011, p. 549).
- Task-specific training teaches stroke survivors new motor skills and activities to perform certain activities in different ways than before their stroke. This training is often described as intense but has been shown to enhance recovery (Good, et al., 2011, p. 549). Furthermore, this training method also engages the patients more as the tasks and actions are relevant to them (Langhorne, et al., 2011, p. 1696).
- Intense repetitive training can also be implemented through the use of robotic devices, such as FES (functional electrical stimulation), and body-weight support training with or without a treadmill (Good, et al., 2011, p. 550).
- Virtual reality, specifically functionally immersive virtual reality, has shown to improve upper limb dexterity, gait, balance and patient independence. Further, the use of VR technology in rehabilitation has been seen to improve patient motivation and compliance (Demeco, et al., 2023, p. 1). Studies comparing traditional rehabilitation programs with that of programs with added VR technology, has shown that the virtual reality groups showed more improvement in arm and trunk movements in terms of motor function and smoothness of movement (Demeco, et al., 2023, p. 12). When coupled with ambulation therapy the VR group showed improved result in their walking and gait (Demeco, et al., 2023, p. 13). Furthermore, functional immersive virtual reality has also been seen to improve post-stroke depressive symptoms and to increase motivation (Demeco, et al., 2023, p. 14).
- Artificial intelligence is being explored with the hope to integrate it into technology that can be used to deliver home exercises at the right levels to stroke survivors (Demeco, et al., 2023, p. 15)
How does Neuromotion incorporate recent research into their stroke therapy program?
TNP (targeted neuroplasticity protocol) is incorporated by:
- Retraining of activities of daily living
- Cognitive retraining - attention, memory, information processing, executive functioning
- Visual/perceptual retraining - visual fields, attention, visual memory & cognition
Bilateral training is incorporated by:
- Balance and gait training
- Integrating aerobic exercise
Constraint-induced movement therapy is incorporated by:
- Strengthening and improving range of motion in the affected limbs
Task-specific training is incorporated by:
- NDT (Neurodevelopmental Treatment) framed treatment utilizing therapeutic handling and task-specific training
- Functional movement training (tasks such as bed mobility, transferring to/from wheelchair, standing, walking, climbing stairs, etc.)
- Performance of household skills (ie. meal prep, housework, etc)
Intense repetitive training is incorporated by:
- Integrating aerobic exercise
- Strength and range of motion training
- Functional Electrical Stimulation training where a client is asked to try and initiate a pedalling movement and a visual response is produced by stimulating muscle contractions that create the pedalling motion.
Neuromotion further covers other aspects of stroke rehabilitation, such as the following:
- Adaptive equipment & home modifications
- Assistance with impacts on speech, voice, and swallowing
- Therapy for challenges with speaking, listening, reading, writing
- Augmentative and alternative communication strategies
- Community reintegration
- Communication confidence building
References used:
Cabral, D. F. et al., 2022. Efficacy of Mechanisms of Neuroplasticity After a Stroke. Restore Neurology Neuroscience, 40(2), pp. 73-84.
Cauraugh, J. H. & Summers, J. J., 2005. Neural Plasticity and Bilateral Movements: A Rehabilitation Approach for Chronic Stroke. Progress in Neurobiology, pp. 309-320.
Dabrowski, J. et al., 2019. Brain Functional Reserve in the Context of Neuroplasticity After Stroke. Neural Plasticity, pp. 1-11.
Demeco, A. et al., 2023. Immersive Virtual Reality in Post-Stroke Rehabilitation: A Systematic Review. Sensors, Volume 23, pp. 1-19.
Good, D. C., Bettermann, K. & Reichwein, R. K., 2011. Stroke Rehabilitation. Continuum Lifelong Learning Neurology, 17(3), pp. 545-567.
Langhorne, P., Bernhardt, J. & Kwakkel, G., 2011. Stroke Rehabilitation. The Lancet, Volume 377, pp. 1693-1702.
Mane, R. M., Chouhan, T. & Gua, C., 2020. BCI for Stroke Rehabilitation: Motor and Beyond. Journal of Neural Engineering, Volume 17, pp. 1-22.
Mohamadpour, M., Whitney, K. & Bergold, P. J., 2019. The Importance of Therapeutic Time Window in the Treatment of Traumatic Brain Injury. Frontiers in Neuroscience, Volume 13, pp. 1-10.
Murphy, S. J. & Werring, D. J., 2020. Stroke: Causes and Clinical Features. Medicine, 48(9), pp. 561-566.
Norman, S. L., Wolpaw, J. R. & Reinkensmeyer, D. J., 2022. Targeting Neuroplasticity to Improve Motor Recovery After Stroke: An Artificial Neural Network Model. Brain Communications, pp. 1-14.
Saway, B. F. et al., 2024. The Evolution of Neuromodulation of Chronic Stroke: From Neuroplasticity Mechanisms to Brain-Computer Interfaces. Neurotherapeutics, Volume 21, pp. 1-7.
Zhou, X. et al., 2021. Mitochondrial Dynamics: A Potential Therapeutic Target for Ischemic Stroke. Frontiers in Aging Neuroscience, Volume 13, pp. 1-14.
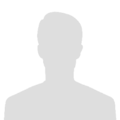